Introduction
The ability to image smaller and smaller samples allows us to see the previously unseen and analyze even the smallest parts of the universe, from viruses to proteins and even the atomic structure of materials.
There are several different techniques available when performing microscopy, each with its own maximum resolution and imaging capabilities. These include light microscopy, electron microscopy, and x-ray microscopy.

Light microscopy has limited spatial resolution due to the diffraction limit of light making it a challenge to image samples below ~200 nm in size. While super-resolution techniques can break this limit, they can often be quite technical, time consuming and require numerous post-processing and deconvolution steps.
An alternative is electron microscopy (EM), which can deliver spatial resolutions at the atomic scale, down to ~0.3 nm. Both scanning (SEM) and transmission (TEM) electron microscopy are frequently used imaging tools, but both techniques require numerous stages of sample prep. In addition, electrons have a low penetration power and scatter within samples, meaning EM samples are typically sliced as thin as 500 nm. Improvements have been made with cryo-EM and this technique is widely used to investigate cellular structures and other biological samples, but even when imaging at cryogenic temperatures EM still results in radiation damage, destruction of sample, and low contrast which leads to resolution issues.
X-ray Microscopy
A complementary technique to visible light and electron microscopy is x-ray microscopy. A range of x-ray energies is used for imaging, including soft x-ray (typical energy <2 keV) and hard x-ray (typical energy >2 keV). Using hard x-rays for imaging can be beneficial as they have high penetration power for thicker specimens and a small wavelength for high spatial resolution (similar to EM and approaching the atomic scale), but using hard x-ray runs into similar issues as EM, namely radiation damage and intensive sample preparation. Soft x-rays have much lower energy and can be used for imaging, for example, whole cells in their hydrated and unstained native state, thick material specimens, or microchemical analysis of light elements, due to the different contrast mechanisms involved. Soft x-rays of certain energies are absorbed by carbon and nitrogen, providing a contrast of certain organic matter vs water (which absorbs x-rays less in the ‘water windows’ of certain x-ray energies from 284 eV to 543 eV).
Soft X-ray Microscopy Techniques
Soft x-ray Tomography
Soft x-ray tomography (SXT) has emerged as a technique that can bridge the gap between visible light microscopy and electron microscopy (see Fig.1) and is often done at cryogenic temperatures (cryo-SXT). Soft x-rays of a specific energy (~500 eV) are passed through a sample and absorbed by carbon-rich structures, and the sample is rotated to generate 2D image slices (tomograms) that can be reconstructed into a high-resolution 3D image without the need for slicing the sample. SXT is often combined with other imaging techniques (such as cryoSIM) to identify fluorescent sample labels, or spectroscopy techniques for elemental analysis. Cryo-SXT is mainly used on biological samples and delivers high-resolution, quantitative information.
An example optical setup for SXT can be seen in Fig.2, along with example tomograms from biological samples.

Scanning Transmission X-ray Microscopy (STXM) and Ptychography
Scanning transmission x-ray microscopy (STXM) is typically performed on thinly sliced samples, where x-rays are focused onto a small spot which is then raster scanned across the sample. The zero-order x-rays transmitted through the sample as well as x-rays scattered off the sample are detected for analysis. In STXM the resolution is limited by the size of the beam spot, usually 30-50 nm.
A similar technique to STXM is ptychography, where a sample is exposed to a focused dot of x-ray energy, and scattered x-rays from the sample are detected in numerous overlapping positions, then computationally constructed into an image. The resolution is the wavelength, meaning that ptychography can achieve higher resolutions than STXM, but some applications only need 30-40 nm resolution.
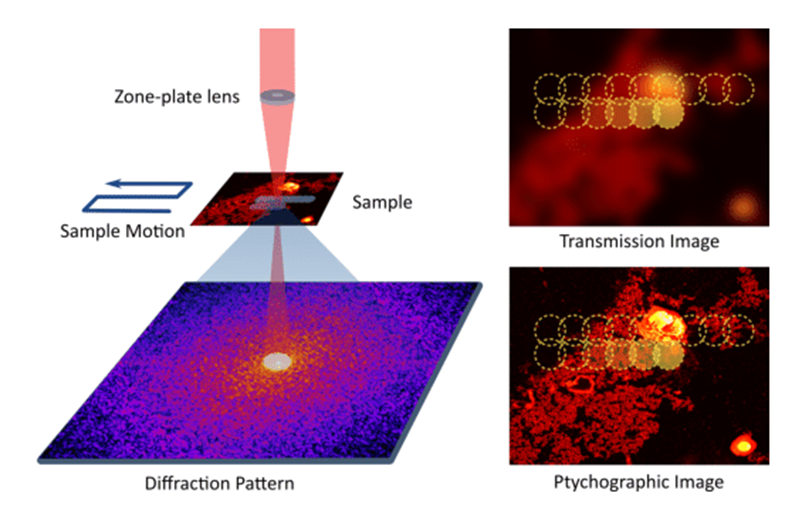
In ptychography the final image is reconstructed by processing many coherent diffraction patterns scattered from the sample and detected by the camera. Ptychography is sensitive to phase changes rather than absorption of x-rays, meaning it can even be used to image transparent samples through phase retrieval processing methods.
If a system can do STXM imaging, it can also be modified to do ptychography. However, STXM is currently much faster than ptychography, as in STXM each pixel is an intensity while in ptychography the resolution is higher and each pixel is an image requiring processing. Improvements in computational processing power mean that ptychography will improve in speed in the future.
Hard x-ray microscopy techniques
Micro Computerized Tomography (µCT)
Micro computerized tomography (µCT) or x-ray microtomography typically uses hard x-rays to achieve higher resolutions (due to hard x-ray’s smaller wavelengths), at the cost of increased sample damage. Many synchrotron beamlines offer µCT imaging due to the ability to obtain high-resolution 3D images, for biological samples as well as electronics, minerals and pharmaceuticals.

Detectors For X-ray Microscopy
X-ray detectors are either direct or indirect and look at a range of x-ray energies that are typically matched to applications. For soft x-ray microscopy techniques such as SXT and ptychography, a detector is usually a 2D direct detection soft x-ray camera featuring a large dynamic range (as x-ray signals can be intense, especially when capturing the zero order beam and diffraction patterns without saturation), a large format sensor (to capture as much data as possible per frame for efficiency – also because and die the scattering is large and the more diffraction orders you capture the better the resolution), a relatively small pixel size (smaller pixels result in higher resolution images, sizes ranging from 5-20 µm are typically used) and the ability to move the camera freely in relation to the sample, which is often rotating and in a vacuum (making this a particular advantage of in-vacuum cameras). Vacuum compatibility is vital for certain soft x-ray detection techniques, as well as the flexibility to have the camera either very close to the sample, or very far away, depending on whether the detectors are being used to analyse transmitted zero-order x-rays or x-rays scattered from the sample. To this end, some select soft x-ray cameras are designed to be entirely in-vacuum, while others are designed to attach to the outside of vacuum chambers.
For hard x-ray it can be more challenging to have direct detect technologies due to the much higher energy beams that could potentially damage the camera electronics. Therefore, indirect detection cameras are often used for hard x-ray, where a scintillator is used between the x-ray photons and the camera. Scintillators convert the x-ray photons into visible light photons, which can be detected by a visible light camera. The other desirable characteristics are similar, with researchers desiring high speeds, large format sensors, and small pixel sizes, all in order to capture as many high-resolution images as possible within a short time.
Conclusion
X-ray microscopy features nanoscale spatial resolution and high penetration and involves diffractive, reflective, and refractive optics. X-ray microscopy techniques are widely used for many different applications and can even image unstained viruses and other typically challenging samples. Further enhancement of resolution is limited by detector pixel size, optical instruments, and x-ray sources.