Advances in Scientific Intensified CCD Camera Technology Enable
New Investigations of Non-Thermal APPJs
“A new gating technology exclusive to the highly advanced PI-MAX4 ICCD camera platform… combines the higher sensitivity of the conventional image intensifiers used in traditional ICCD cameras with the ability to deliver <500 picosecond resolution.”
Introduction
Non-thermal ‘atmospheric pressure plasma jets’ (APPJs) hold great promise for innovative applications in material processing and biomedicine/healthcare because of their ability to generate plasmas that are not confined between electrodes1. Produced using one of several different experimental setups (e.g., a number of jet configurations are based on dielectric barrier discharge, or DBD), fed with pure rare gases (helium, neon, or argon), and excited by pulsed high voltages, all non-thermal APPJs exhibit a unique feature: they are not continuous media but actually consist of ‘plasma bullets’ traveling at high velocity in ambient air1.
The growing utilization of these low-temperature APPJs during the past several years has led to the desire to better understand the physical phenomenon of the plasma bullets (spatio-temporally localized luminous effects) in the effluent of the jets2. Imaging studies of a cold APPJ in an argon atmosphere performed with a high-speed intensified CCD (ICCD) camera, for example, have shown that plasma bullets can have a propagation velocity of up to 20 kilometers per second2. The appearance both of the plasma bullets as well as the spatio-temporal evolution of the generation zone of metastable argon atoms can be explained by the effect of a self-propagating ionization front2.
It is important to note that non-thermal APPJs can propagate over rather long distances; however, their diameters are limited to only a few millimeters1. While this spatial coverage is sufficient for small-scale applications, a more efficient solution for large-scale applications is to organize several plasma jets in 1D or 2D arrays operating simultaneously from a unique power supply1.
This application note will provide an overview of non-thermal APPJ experimental setups for single-jet and multiple-jet studies in addition to the newest relevant imaging technology.
Single-Jet Studies
A typical non-thermal APPJ experimental setup comprises a glass tube with two external electrodes of certain length (along the axis of the tube) with a gap between them. Plasma is formed in the flowing rare gas (often helium). Plasma packages travel within the tube and outside in the air, where they form bullets even though there is no external field. The apparent speed of visible plasma packages, ionization fronts, and plasma bullets is much greater than the speed of the flowing feed gas3.
ICCD cameras enable researchers to observe the development of plasma bullets in cold APPJs by tracing the formation of plasma packages as a discharge close to the instantaneous cathode, following their motion between and inside the electrodes up to their emergence at the edge of the glass tube, and then capturing the formation of a plasma bullet. Inside both electrodes, plasma is concentrated close to the walls and is bright, while outside it is located at the axis3. See Figure 1.
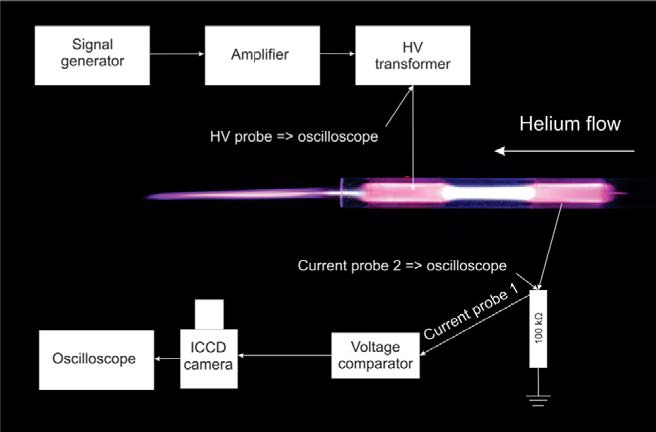
Low-temperature APPJs offer controllability of a variety of agents (e.g., radicals, ions, UV, and electric fields) and do not cause thermal damage to heat-sensitive biological systems, which makes them suitable for biomedical applications such as sterilization, treatment of mammalian and cancerous cells, blood coagulation, wound healing, and dental treatments4.
The photograph in Figure 2, for example, shows a plasma generated in the root canal of a tooth using the non-thermal APPJ device depicted in Figure 3.
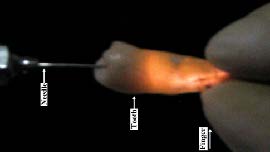

Because of the narrow channel geometry of a root canal, which typically has a length of a few centimeters and a diameter of one millimeter or less, the plasma generated by a traditional jet is not efficient enough to deliver reactive agents into the root canal for disinfection. To achieve better killing efficacy, a plasma needs to be generated inside the root canal, whereupon reactive agents, including short-lifetime species such as charged particles, could play a role in killing bacteria5. Utilizing the device shown in Figure 3, a cold plasma could be generated inside a root canal (Figure 2).
Multiple-Jet Studies
Generating multiple non-thermal APPJs in order to cover larger treatment areas has recently become desirable; however, since the interaction between neighboring jets cannot be avoided it is therefore imperative to elucidate the physical processes that occur between jets1. It could also be of interest to produce an interaction between two or more of these small-diameter jets impacting the same location of a target to increase the deposited dose of plasma, or to finely tune the composition of the reactive species using a different gas mixture feed in each jet1.
Material processing and chemical decontamination, as well as biomedicine/healthcare, are among the fields that could benefit from the use of multiple low-temperature APPJs. Figure 4 shows an experimental setup utilized to perform a comprehensive study of the physical properties of the interaction between two microplasma jets produced in helium and counter-propagating in ambient air1.

In this study, the spatio-temporal evolution of plasma emission was investigated using an advanced ICCD camera (Teledyne Princeton Instruments PI-MAX®3:1024i) that permits exposure times as short as 0.39 nanoseconds with a fully integrated high-voltage controller. Spectroscopic measurements were made with a 750 mm focal length spectrometer (Princeton Instruments Acton SP 2750i) equipped with three different gratings, allowing the researchers to study plasma emissions in the wavelength range from 190 to 900 nanometers.
Figure 5 presents two ‘snapshots’ (exposure times: 5 nanoseconds) of the microplasma jets propagating in ambient air as they arrived in a turbulent area.

A fascinating feature of the interaction between the two counter-propagating plasma jets is the sudden occurrence of a secondary glow discharge at later times (see Figure 6).
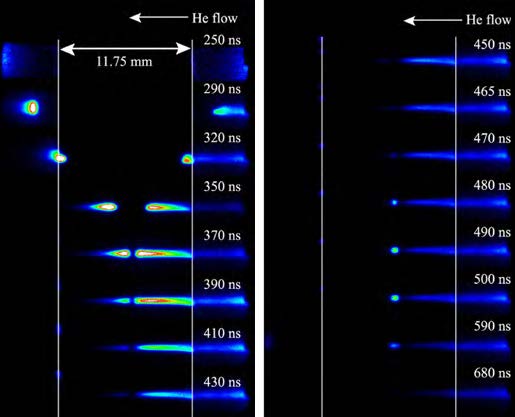
of the propagation of two counterpropagating
microplasma jets acquired with a 16-bit, precision-gated Princeton Instruments ICCD camera. Helium was fed only through the device on the right, so as to avoid hydrodynamic instabilities1. Courtesy of Dr. Vincent Puech.
The body of data collected reveals that the plasma bullets, which constitute the jets, interact with each other from the time they leave the nozzle of their respective devices. This interaction results in a decrease in the velocity of both plasma bullets. More important, the ignition of a ‘secondary’ discharge, a pink glow, suddenly appears in the small space between the jets where no bullet propagation occurs (Figure 7).

Based on current measurement analysis, correlated with optical emission spectroscopy and high-speed digital photography observations, it is suggested that this transient pink glow is attributable to a secondary negative glow induced by the sudden reversal of the potential of the plasma columns, instantaneously correlated with the voltage reversal of the DBD electrodes, while the floating potential of the previously dark zone remains almost unchanged1.
New Imaging Technology for Time-Resolved APPJ Studies
Recently, Princeton Instruments introduced a new gating technology exclusive to the highly advanced PI-MAX4 ICCD camera platform that combines the higher sensitivity of the conventional image intensifiers used in traditional ICCD cameras with the ability to deliver <500 picosecond resolution.
By utilizing state-of-the-art electronics and fiberoptically bonding the intensifier to the CCD sensor, this new picosecond gating technology enables PI-MAX4 cameras (see Figure 8) to gate conventional image intensifiers, which normally achieve ~2 to 3 nanosecond gating, at <500 picoseconds without sacrificing quantum efficiency (QE).
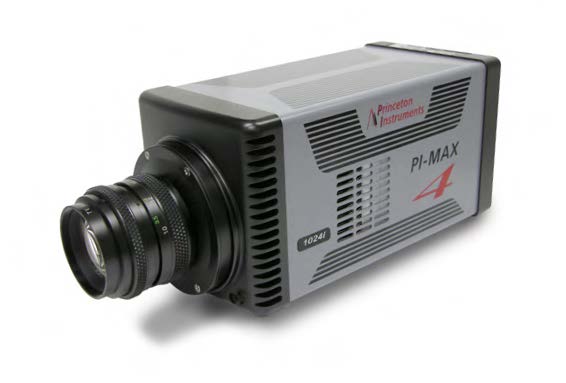
An integrated programmable timing generator, SuperSynchro, built into these cameras makes them perfect for single- and multiple-jet studies. Furthermore, the latest Princeton Instruments LightField® 64-bit data acquisition software, available as an option, affords complete control over all PI-MAX4 hardware features via an exceptionally intuitive user interface. LightField provides automatic defect correction, precision exposure control, and a host of innovative functions for easy capture and export of non-thermal APPJ imaging and spectral data.
Summary
The Teledyne Princeton Instruments PI-MAX4 family, the new benchmark for ICCD camera performance, combines the advantages of picosecond gating with the high QE of conventional image intensifiers fiberoptically coupled to scientific-grade sensors. These cameras are well suited for the latest studies of non-thermal APPJs as well as numerous other time-resolved imaging and spectroscopy applications.
Low-temperature APPJs are quickly gaining popularity in areas such as biomedicine/healthcare, material processing, and chemical decontamination. Myriad single-jet and multiple-jet setups each provide their own distinct advantages for various practical applications across these diverse realms. As researchers’ efforts to characterize and develop non-thermal APPJs become even more refined, highly advanced scientific ICCD cameras will play an increasingly critical role in such endeavors.
Achknowledgements
Princeton Instruments wishes to thank Dr. Vincent Puech (Laboratoire de Physique des Gaz et des Plasmas, CNRS & Univ. Paris-Sud, Orsay, France) for his contributions to this application note.
Resources
For more information about the Princeton Instruments PI-MAX4 family of cameras, please visit: https://www.princetoninstruments.com/products/pi-max-family/pi-max
References
[1] C. Douat et al., “Dynamics of colliding microplasma jets,” Plasma Sources Sci. Technol. 21 034010 (2012).
[2] R. Bussiahn et al., “Spatially and temporally resolved measurements of argon metastable atoms in the effluent of a cold atmospheric pressure plasma jet,” J. Phys. D: Appl. Phys. 43 165201 (2010).
[3} N. Puač et al., “Time resolved optical emission images of an atmospheric pressure plasma jet with transparent electrodes,” Appl. Phys. Lett. 101 024103 (2012).
[4] G.Y. Park et al., “Atmospheric-pressure plasma sources for biomedical applications,” Plasma Sources Sci. Technol. 21 043001 (2012).
[5] X. Lu et al., “On atmospheric-pressure non-equilibrium plasma jets and plasma bullets,” Plasma Sources Sci. Technol. 21 034005 (2012).