Introduction
Laser-cooled ions in linear Paul traps are quantum systems with remarkable properties. Trapped ions offer an unprecedented degree of preparation and control of their parameters, can be cooled to the ground state, and can be coupled to engineered reservoirs. For these reasons, they have played a prominent role in the experimental study of quantum computation and information processing applications. They are also invaluable tools for the investigation of quantum thermodynamics.1
Today, researchers in many fields use trapped ions to perform experiments that enable the investigation of quantum phenomena. The list of applications employing trapped ions is growing quickly and this type of work likely holds the key to major advances in such diverse areas as quantum computing, quantum cryptography, and light-based telecommunications. The present application note will focus on the research being conducted jointly by scientists from two universities in Germany (Mainz, Augsburg). This group of scientists recently proposed an experimental scheme to realize a nano-heat engine with a single ion.1
Quantum Heat Engines
The fundamental question that the aforementioned group of scientists seeks to address is whether commonly realized macro-heat engines like those utilized by automobiles, which convert thermal energy into mechanical work, can be scaled down to the single-particle level while retaining the same working principles. The scientists have analyzed an experimental scheme for a nano-heat engine using a single laser-cooled ion as working gas.
In experiments performed at the Institut für Quantenphysik, Universität Mainz, the team has implemented an Otto cycle, the basis of the four-stroke car engine, by confining the cold ion in a linear Paul trap with a tapered geometry (see Figure 1) and coupling it to a pair of engineered reservoirs.


Figure 1. Left: A linear Paul trap for the singleion heat engine. Its tapered geometry allows the coupling of radial states to the axial oscillation.2 Right: Experiment setup showing the Paul trap in a vacuum chamber. Photos courtesy of Prof. Dr. Ferdinand Schmidt-Kaler, Dr. Kilian Singer, Johannes Rossnagel, and Georg Jacob (Institut für Quantenphysik, Universität Mainz)
The two heat baths alternately heat and cool the radial thermal state of the ion. The change of radial temperatures is converted into axial movement — and thus into usable work. By repeating this cycle resonantly with the axial eigenfrequency at 100 kHz, large coherent amplitudes along the trap axis are accumulated.2 A classic analog is presented in Figure 2.

Singer, Johannes Rossnagel, and Georg Jacob (Institut für Quantenphysik, Universität Mainz).
The German scientists have analytically determined the quantum efficiency of the single-ion heat engine at maximum power in various regimes. They have also performed Monte Carlo simulations of the engine that not only demonstrate its feasibility but its ability to operate at maximum efficiency of 30% under realistic conditions.1
Although micro-heat engines have been constructed employing various types of systems, a quantum heat engine had never been built. Encouraged by their numerical simulations and analysis, however, the scientists in Germany have now fabricated a single-atom engine.3
Experiment Imaging
In their experiments, a single calcium ion is trapped in the electromagnetic field of a special Paul trap and cooled via laser to a temperature of 1 mK. To drive the ion in a heat engine cycle, the scientists alternately heat and cool the ion by applying electric noise and laser cooling.
The figure of merit for the experimental demonstration of the single-ion heat engine is the work produced as a function of the temperatures of the heat baths. As the work produced is directly converted into motion in the form of an oscillation at 100 kHz, a precise observation of the latter is crucial.
In order to observe the oscillation of the ion at 100 kHz, the scientists employ an emICCD camera. Exposure times of only 0.5 μsec allow the ultra-high-sensitivity imaging system to resolve the ion motion precisely in both space and time (see Figure 3).
It is the fast gating ability of the emICCD camera that has enabled the scientists to demonstrate the functionality and performance of the single-ion heat engine. This will lead to a further understanding of the thermodynamics of single particles.

Enabling Technology
The PI-MAX4:1024EMB emICCD camera (see Figure 4) seamlessly combines the rapid gating capabilities of an image intensifier and the excellent linearity of a back-illuminated, frame-transfer, 1024 x 1024 EMCCD detector in order to deliver quantitative, ultra-high-sensitivity performance for applications executed on nano- and picosecond timescales.

This fiberoptically bonded Princeton Instruments PI-MAX®4 camera system provides <500 psec gate widths using standard fast-gate intensifiers while preserving quantum efficiency. Its integrated SuperSynchro timing generator allows camera users to set gate pulse widths and delays under GUI software control, and significantly reduces the inherent insertion delay (~27 nsec).
Complete control over all PI-MAX4:1024EMB hardware features is simple with the latest version of Princeton Instruments’ LightField® data acquisition software (available as an option). Precision intensifier gating control and gate delays, as well as a host of novel functions for easy capture and export of image data, are provided via the exceptionally intuitive LightField user interface.
The PI-MAX4:1024EMB uses a high-bandwidth (125 MB/sec or 1000 Mbps) GigE data interface to afford camera users real-time image transmission. This interface supports remote operation from more than 50 meters away.
Alternatively, research that does not require gating can benefit from advanced high-speed EMCCD technology such as that implemented in Princeton Instruments’ ProEM-HS cameras (see Figure 5). The ProEM® “HS” series offers a special kinetics readout mode that increases temporal resolution by illuminating a small portion of the sensor and then capturing and shifting a series of sub-frames in microseconds. These high-sensitivity, back-illuminated EMCCD cameras are engineered to keep up with the latest high-repetition-rate lasers.
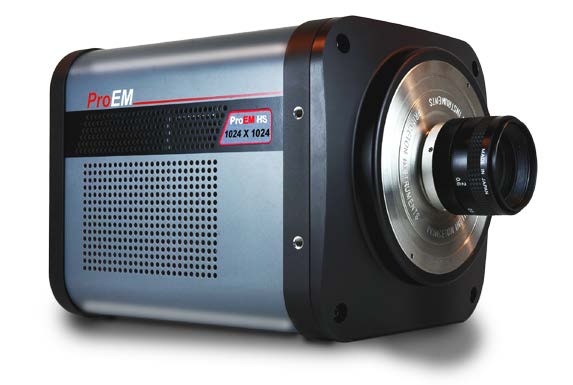
Future Trends
As myriad technologies, devices, and systems march inexorably toward the ultimate limit of single quantum particles, experimental studies will become more challenging and complex. Accordingly, next-generation scientific cameras must be able to provide the outstanding sensitivity and speed demanded by such investigations. The PI-MAX4:1024EMB and ProEM-HS cameras mentioned herein represent the leading edge of high-performance quantitative imaging capabilities for research utilizing trapped ions
References
- Abah O., Rossnagel J., Jacob G., Deffner S., Schmidt-Kaler F., Singer K., and Lutz E. Single-ion heat engine at maximum power. Phys. Rev. Lett. 109, 203006 (2012). DOI: 10.1103/PhysRevLett.109.203006
- https://www.quantenbit.physik.uni-mainz.de/index.wt/index.wt/#/research/thermo (accessed online June 2015)
- Rossnagel J., Abah O., Schmidt-Kaler F., Singer K., and Lutz E. Nanoscale heat engine beyond the Carnot limit. Phys. Rev. Lett. 112, 030602 (2014). DOI: 10.1103/ PhysRevLett.112.030602